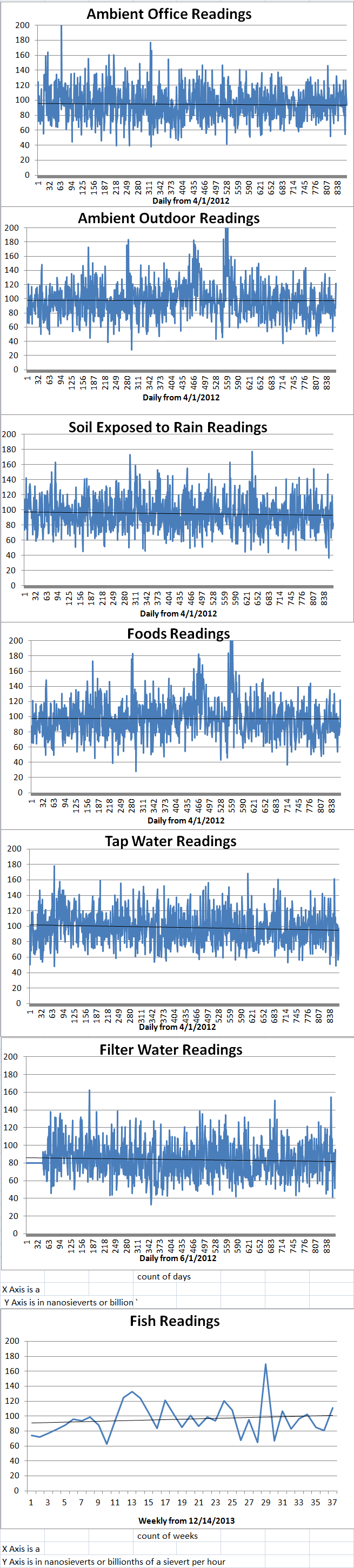
Blog
-
Geiger Readings for November 3, 2014
Ambient office = 103 nanosieverts per hourAmbient outside = 126 nanosieverts per hourSoil exposed to rain water = 112 nanosieverts per hourCrimini mushroom from Central Market = 74 nanosieverts per hourTap water = 89 nanosieverts per hourFiltered water = 77 nanosieverts per hour -
Geiger Readings for November 2, 2014
Ambient office = 94 nanosieverts per hourAmbient outside = 86 nanosieverts per hourSoil exposed to rain water = 82 nanosieverts per hourGrape from Central Market = 122 nanosieverts per hourTap water = 98 nanosieverts per hourFiltered water = 95 nanosieverts per hour -
Radiation News Roundup November 1, 2014
-
Geiger Readings for November 1, 2014
Ambient office = 105 nanosieverts per hourAmbient outside = 115 nanosieverts per hourSoil exposed to rain water = 75 nanosieverts per hourRedleaf lettuce from Central Market = 86 nanosieverts per hourTap water = 66 nanosieverts per hourFiltered water = 62 nanosieverts per hourRockfish – Caught in USA = 111 nanosieverts per hour -
Malaysian Physicians for Social Responsibility Fight Malaysian Nuclear Power Plans
I have blogged before about the thrust of nations which export nuclear technology to sell nuclear reactors to developing nations. I recently read about that the United States has offered to help Malaysia build a nuclear power plant. In 2009, the Malaysian government presented its plan for nuclear power claiming that nuclear power was inexpensive, clean and safe.
The Malaysian Physicians for Social Responsibility (MPSR) are not happy about the project. “We have been working to convince the government that such a project is not a wise or viable energy option since it carries inherent risks and is not affordable, economically viable, socially acceptable and environmentally sound.”
The MPSR believe that “the cost of electricity generated by a nuclear power plant is far higher than electricity from fossil fuels or renewable energy.” They quote Moody’s Corporate Finances that rates the relative risk of investments about the cost of electricity from nuclear power. Moody’s estimated that the when nuclear power generates a kilowatt, the cost of that kilowatt is almost three hundred percent higher than a kilowatt generated by wind power and about one hundred and fifty percent higher than a solar energy kilowatt. (Both wind and solar power keep getting cheaper as nuclear power keeps getting more expensive.)
The MPSR also cite a 2014 World Nuclear Industry Status Report that projects that the adoption of renewable energy sources will increase at a rapid pace as the investment in renewables far surpasses the future investment in nuclear power plants and fossil fuel plants.
The MPSR mentions that Greenpeace has identified almost a hundred minor nuclear accidents across the globe between 1952 and 2009. Greenpeace claims that any of those accidents could have easily escalated into a major accident that would threaten the environment and human health. The horrible nuclear disaster at Fukushima vividly displays just what can happen when a conjunction of factors destroys a nuclear power plant.
The MPSR says that the “most dangerous and unacceptable” feature of the use of nuclear reactors to generate electricity is the production of radioactive waste, some of which can remain radioactive and dangerous for thousands of years. While there has been some effort to create underground repositories where such waste could be safely stored, there is no global solution to disposing of the hundreds of thousands of tons of spent nuclear fuel that have been generated by nuclear power plants.
The MPSR complains that there is no compelling evidence that nuclear power is safe, clean or cheap. They say that there is little public support for the project, that there has not been a genuine dialog, debate or consultation with the Malaysian public about the project. The MPSR points out that other nations are phasing out nuclear power and calls on the Malaysian government to take into account the dangers of nuclear power and cancel the project.
I sincerely hope that other developing nations can mount serious opposition to the current hard marketing push of the nations that export nuclear technology.
-
Geiger Readings for October 31, 2014
Ambient office = 127 nanosieverts per hourAmbient outside = 133 nanosieverts per hourSoil exposed to rain water = 119 nanosieverts per hourRomaine lettuce from Central Market = 88 nanosieverts per hourTap water = 56 nanosieverts per hourFiltered water = 52 nanosieverts per hour -
Nuclear Reactors 176 – Agressive Thyroid Cancers in Balarus Connected to Chernobyl Disaster
I have often blogged about adverse health effects of radiation. One of the big problems with exposure to radiation is that there are many other factors that influence health impact. In addition, the radiation is invisible which makes it harder to know about possible exposure. And it can take years for the appearance of any symptoms from radiation poisoning.
I have also blogged about the terrible accident at the Chernobyl Nuclear Power Plant, Ukraine in 1986 while it was under the control of the Soviet Union. “An explosion and fire released large quantities of radioactive particles into the atmosphere, which spread over much of the western USSR and Europe.” There was a recent article about wild boars in Germany with flesh too radioactive to eat. The hogs were contaminated while rooting in ground containing radioactive materials from Chernobyl from the accident that occured almost forty years ago.
Now a medical study by members of the d has discovered that exposure to radioactive iodine released by Chernobyl was associated with an especially aggressive form of thyroid cancer. The survey was conducted on twelve thousand people who were children or adolescents in Belarus when the Chernobyl accident occured. The study was based on diagnoses of thyroid cancer in the twenty years following Chernobyl. As the estimated level of radiation exposure increased, the aggressiveness of the tumors increased. The conclusions of the study suggest that many children and adolescents in the area of the Fukushima March 2011 nuclear disaster are at risk for especially aggressive forms of thyroid cancer. There have been reports of increases in childhood thyroid disease in the vicinity of the Fukushima nuclear power plant following the disaster.
The study also found that exposure to radioactive iodine can cause a wide spectrum of thyroid diseases from the relatively harmless to the very dangerous. In any case, the treatment for any serious thyroid illnesses is to surgically remove the thyroid and to have the patient take pills to replace the hormones produced by a healthy thyroid gland. Taking these supplement pills for a lifetime can be expensive and complicated. Thyroid cancer is usually rare among children and among adults, on average, there will be around thirteen cases per one hundred thousand people. However, there were one hundred and fifty eight thyroid cancers in the sample of almost twelve thousand people in Belarus. This is about one hundred times the normal rate of thyroid cancer. Higher radiation doses were associated with more aggressive cancers that spread to lymphatic vessels.
I posted recently about accepted radiation release levels for operating nuclear power plants in the United States. The companies operating the power reactor report their averages emissions for the year and, as long as the average does not exceed the limit set by the NRC, the reactors were deemed to be operating safely. However, it turns out that when the reluctant companies were forced to release their hour by hour emissions, there was a huge spike in emissions when the reactor was opened for refueling. Among the radioactive materials releases in such spikes was a radioactive isotope of iodine. These is evidence of an increase in childhood leukemia and other health problems downwind of these plants but it was hard to pinpoint because the period of exposure iss brief and most dangerous to pregnant women.
A recent metastudy of naturally occurring background radiation levels and health revealed that there is a close relationship between natural levels of background radiation and health problems related to radiation exposure. In other words, it appears that there is no “safe” level of radiation. Starting from zero background radiation, every increase in background radiation is associated with an increase in cancer, birth defects and other health problems. Bottom line, even nuclear power plants operating within acceptable parameters are not “safe” to those who live nearby.
-
Radiation News Roundup October 30, 2014
Mutations in plants being reported after chronic Fukushima exposure. enenews.com
Further legal challenges have been filed against the US Nuclear Regulatory Commission (NRC) ruling on storage of used nuclear fuel. world-nuclear-news.org
Belgium’s nuclear regulator has outlined a two-step review process for Electrabel’s safety case report for the restart of the Doel 3 and Tihange 2 reactors. world-nuclear-news.org